Paper Chemistry in the Digital Age
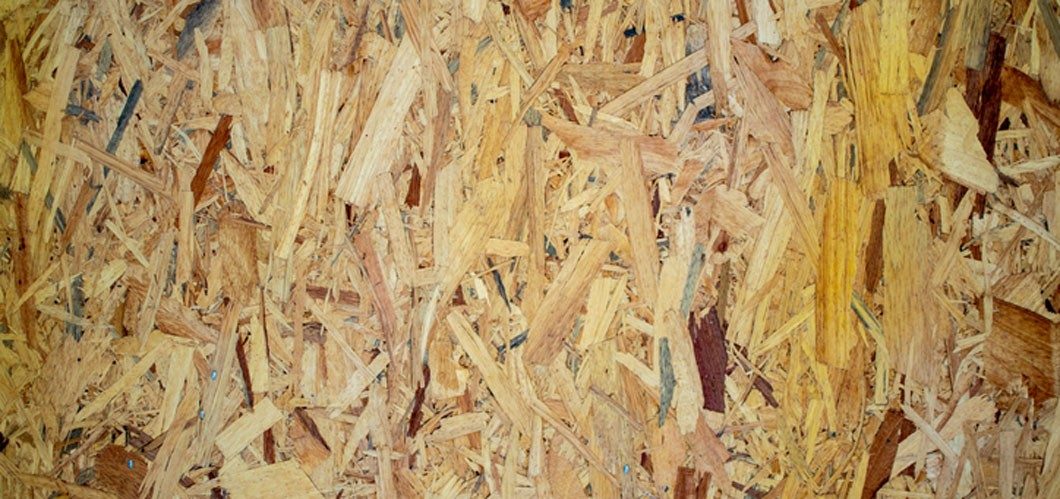
More and more in the digital age, e-books and electronic communication are replacing printed books and hand-written letters. Yet paper in its physical form is still everywhere. You can find it in nearly every room of your home (e.g., toilet paper in the bathroom), at school, at your workplace, in your car (the manual that explains all the lights on the dashboard), and in places you frequent (restaurant menus, museum maps, and transit booklets).
In fact, you might be hard-pressed to find a place without paper, except maybe the forest – where the raw materials that create paper come from.
Although paper is indispensable in everyday life, you may not be familiar with the distinct properties that make it the chameleon of materials. It can be clean and bright enough for us to write notes on, tough enough to form shipping boxes, and soft and absorbent enough to wipe our noses with.
Remarkably, considering the vast array of paper’s useful characteristics, the composition of most paper products is essentially the same: fibers derived from trees.
Strong, but not too strong
At the core of paper’s versatility are the fibers. They are strong and flexible and can bond to each other. Most paper products that we encounter daily, such as printer paper, brown cardboard boxes, paper towels, and facial tissues, are assemblies of fibers that are almost entirely composed of a sugar called holocellulose. Holocellulose is made up of long-chain polymer cellulose and a variety of hemicelluloses, which are shorter and may be branched.
The cellulose in paper is responsible for its unique and varied properties. Cellulose, the most abundant natural polymer, also gives distant cousins of paper, such as the woven cotton textiles that are used to make T-shirts and blue jeans, the durability, comfort, and water absorbency that we value in clothing.
To further understand how fibers derived from trees are used in paper, we need to look at the structure of fibers. Wood fibers generally come in two lengths. Longer fibers from softwoods, which come from evergreens, are 3–5 millimeters long. The longer fibers are used to reinforce paper so that it can withstand being pulled apart or torn. Hardwood fibers, which come from deciduous trees—the type that lose their leaves every year—are typically much shorter, about 1.2 millimeters long on average. These shorter fibers are used to fill in gaps and make paper smooth. Both hardwood and softwood fibers usually have widths less than that of a human hair (about 50 micrometers).
Closer observation reveals how the chemistry of wood fibers’ microstructure is important for paper’s strength and flexibility. First, the long polymeric chains of cellulose, which have about 10,000 units of glucose (C6H12O6), hydrogen-bond to each other to form strong, rigid crystalline regions. These structured components connect to unstructured, flexible regions.
Within trees and other plants, microfibrils—long, skinny strands of connected cellulose—form in the plants’ cell walls. Many layers of microfibrils are wrapped around an open center, called the lumen (Fig. 1).

Because of its many polar hydroxyl (–OH) groups, cellulose is hydrophilic, so water is attracted and can be absorbed between the microfibrils. This makes the fiber wall softer and even more flexible (think of how dry spaghetti softens in boiling water) (Fig. 2a).
In papermaking, this flexibility allows the fibers to be flattened into ribbon-like structures as the paper is pressed. The fiber wall can also easily conform to the shapes of other fibers where they cross each other, further increasing the contact area between the fibers. This enables hydrogen bonds to form between cellulose chains as the newly made paper dries (Fig. 2b).
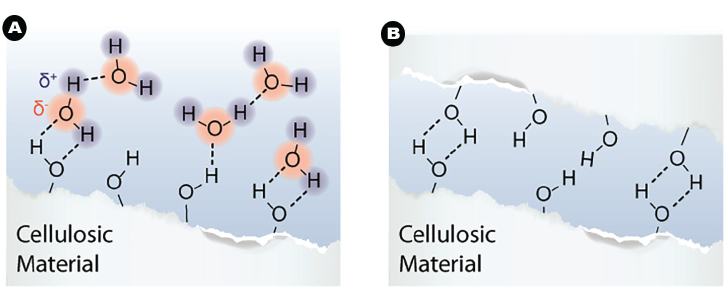
For some paper products, like toilet paper, hydrogen bonding between cellulose chains provides all the strength that’s required for the final product. After use, these products should come apart easily, and because cellulose is hydrophilic, water can do the trick. It gets between the cellulose chains and causes the hydrogen bonds between cellulose molecules to break as new hydrogen bonds form between the cellulose and water. You can see this in action when toilet paper is immersed in water. Its structure breaks apart, and the fibers disperse.
Such vulnerability to water comes in handy when we want to recycle other types of paper. Recycling facilities simply place recovered paper into a hydrapulper (a big blender) filled with water. The hydrapulper vigorously mixes the paper (e.g., newspaper, corrugated containers) with water, and the fibers easily separate from each other, so they can be cleaned and reused.
Fillers and Fibers
Thanks to sizing agents, we have printer paper that won’t fall apart if a bit of rain or a glass of water spills on it. But in addition to varying degrees of durability, we’ve come to expect printer paper to be smooth and bright white—not the color of raw wood.
To achieve these requirements for texture and appearance, manufacturers replace as much as 25% of the wood fiber with a filler composed of calcium carbonate (CaCO3) particles, which can be prepared synthetically or mined from the earth as marble or chalk. The filler particles are very small, with a diameter about 1/10 the width of a wood fiber. This small size increases the scattering of light to make paper appear whiter. Even with this extra load, paper maintains much of its strength and resistance to damage from water.
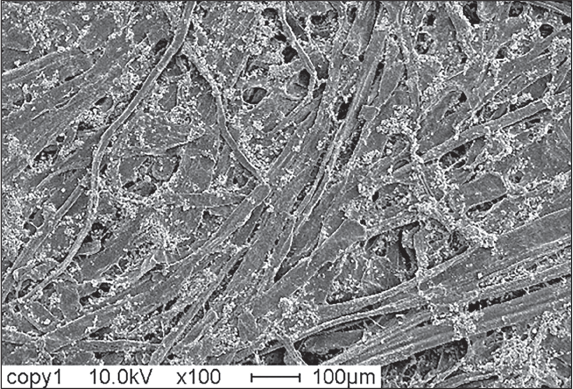
For sturdier stuff
Unlike toilet paper, products like mailing envelopes, shipping boxes, and office paper need to be able to stand up to rain and the occasional splash of spilled water. Such products should also be recyclable.
To add some resistance to the effects of water on hydrogen bonds between the cellulose chains in paper, the bonds need protection. This is where sizing agents come in. Sizing agents are organic compounds that are hydrophilic on one end and hydrophobic on the other. When they are added to the wood fibers, the hydrophilic ends covalently bond with cellulose during the drying process. The hydrophobic ends face outward toward the surface of the paper and help slow the absorption of water.
In some cases, paper manufacturers also apply a starch solution with micrometer-size hydrophobic particles to the surface of a paper. The starch forms hydrogen bonds with the cellulose, seals some of the pores, and coats some of the fiber–fiber bonds. The fine hydrophobic particulates slow the spreading of water on the fiber surfaces.
This X-ray 3D microscopic video of office copy paper with a diameter of 1.4 mm shows how the hollow fibers have been collapsed in the pressing and drying process to create a smooth flat surface and a structure that is much denser than paper towel. The pape The paper has been calendered (passed between two solid rolls under pressure) to make the surfaces smoother and ensure a uniform thickness, which is ideal for fine print quality. The dense structure is also stronger as there is more fiber-fiber bonding. Looking carefully, one can identify the long and wide softwood fibers, and the short and narrow hardwood fibers.
Although papers treated with sizing agents are stronger than facial and toilet tissue, they are still readily recyclable under the right conditions. The process commonly involves breaking the paper down into smaller pieces in a machine called a repulper. Additional equipment separates out the remaining debris, such as staples or plastic. The fibers then pass through a refiner, which bends the fibers and reopens the fiber cell walls. This allows fiber to swell and become more flexible. The fibers can then re-form bonds, as they did when they were new.
Now that we’ve figured out how to make paper more water-resistant while maintaining its recyclability, let’s look at a paper product designed to be the opposite of water-resistant. Paper towels need to be strong and absorb liquids quickly. To combine these traits into one product, manufacturers add chemicals called polymeric cross-linkers. These agents form covalent bonds between the cellulosic fibers, so that water can enter the structure without separating the fibers from each other. However, the amount of bonding in paper towels is limited. This keeps the density low, so lots of internal spaces are available for liquids to flow into.
This X-ray 3D microscopic video of a 1.6 mm x 1.6 mm region of paper towel shows clearly defined fibers. Unlike dense copy paper, the complex structure has densified and bulked regions due to creping and drying processes, which provide strength, flexibility, and absorptivity. The hollow fibers have collapsed into ribbons. The wide fibers are from softwood trees and the short thin fibers are from hardwood trees. The small holes in the fibers, called border pits, are regions through which water was passed when the fibers were in the wood.
So, the next time you wipe up a spill with a paper towel, use a cardboard box, or take a test on paper, you can think about the chemistry that went into creating each of those useful products.
More about paper chemistry
Articles and Activities
Chemists Celebrate Earth Week
Infographic
Paper currency
SELECTED REFERENCES
Paper: Science and Sustainability. International Paper Company: https://www.hammermill.com/paper-is-power-free-school-curriculum [accessed Feb 2019]
Smook, G. Handbook for Pulp and Paper Technologists. 4th Edition. TAPPI Press: Peachtree Corners, Ga., 2016.
Scott, W. Principles of Wet End Chemistry. TAPPI Press: Peachtree Corners, Ga. 1996.
This story is adapted from "Celebrating Paper: Why Paper Thrives in the Digital Age," ChemMatters magazine, April 2019.